A – Z Microbial Science
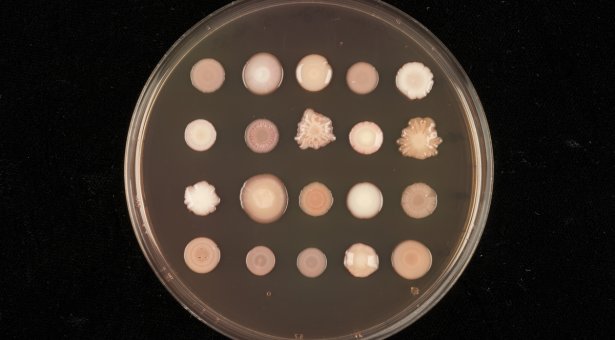
Microbial science may look at the microscopic, but the breadth of microbial research being done here at the John Innes Centre is massive, so here’s an A-Z covering some of it.
A – Antimicrobial Resistance and Antibiotics
Antimicrobial Resistance (AMR) is the ability of a microbe (e.g. a bacterium) to survive antibiotic treatment. AMR also covers other microorganisms like fungi, which can be problematic in terms of human infections.
We recently spoke to Professor Tony Maxwell, who told us; “Simply put, we are running out of antibiotics. We have plenty of antibiotics in our armoury after all, the problem is that a significant number of these are meeting a significant level of resistance. It is a problem in the UK, particularly in hospitals, but it is a major problem across the globe, where some hospitals have a resistance rate of close to 100% to certain antibiotics, which means that a patient presenting at a hospital may not be treatable with the normal course of antibiotics.
The good thing about antibiotics is that they are small(ish) chemical molecules that can generally be synthesised, produced and tolerated by humans. There are a number of alternatives to antibiotics such as vaccines and phage therapy, which should also be investigated further. However, they all take time, up to a decade or two, to go from lab through clinical and non-clinical trials, before they can be used.
Public-funded research and development is not a straightforward solution and it is not a cheap solution, but it probably is cheaper than paying pharmaceutical companies to do the same thing. My concern is that the time to do this is now, not in five or 10 years’ time; if we wait, it could be too late.”
B – Biochemical pathways
Biochemical pathways are linked series of chemical reactions, occurring within a cell which are required for the maintenance of homeostasis.
C – Caulobacter crescentus
An unassuming crescent moon shaped bacterium offers insight into how DNA is organised inside cells; Caulobacter crescentus.
Understanding the spatial arrangement of DNA in bacterial cells and the significance of this for processing and maintaining the information it encodes is the focus of Dr Tung Le’s research.
Recent technological developments, such as chromosome conformation capture assay (also known as Hi-C) make visualising DNA organisation possible. Scientists are now able to capture a snapshot of the spatial organisation of DNA inside cells.
D – David Hopwood
Streptomyces bacteria are today recognised as globally important industrial microbes, producers of most of the world’s antibiotics.
But 60 years ago, understanding of what Streptomyces were, or how they functioned was limited and it was not until the 1950s that they were identified as bacteria and not fungi moulds or a hybrid between the two as previously debated.
The change in understanding is due in no small part to the work of one man, Professor Sir David Hopwood.
E – Effectors
Plant pathogens are a serious threat to agriculture and to global food security, causing diverse crop diseases which lead to extensive annual yield losses.
Production of effector proteins by pathogens, to manipulate host cellular processes, is central to their success. An understanding of fundamental effector biology is key to addressing the threat posed by these pathogens.
Postgraduate Researcher Juan Carlos De la Concepcion is one of a number of our scientists who are working towards understanding the mechanistic basis of pathogen recognition by plant immune receptors and using that information Juan hopes to contribute to the ultimate goal of engineering new disease resistance in crops.
F – Fungus
Microbial science or Microbiology is the study of microorganisms, a diverse group of generally tiny life-forms that include fungi, as well as; bacteria, viruses, archaea, algae, and protozoa.
We aim to understand the molecular dialogue between plants and microbes, establishing how they communicate with each other and how they have evolved in relation to one another. Understanding and being able to influence these interactions is crucial to increasing future crop productivity.
Professor James Brown, Head of Crop Genetics at the John Innes Centre said “We know that the fungus is a very aggressive pathogen in Europe, and we know that on ash species native to East Asia it reproduces prolifically but is not a destructive pathogen there. So, one of the questions is ‘What is likely to happen to this disease in Europe and Britain specifically?’ And to answer that we need to understand how natural selection operates in the fungus.”
G – Geosmin
Geosmin is the molecule which gives soil its distinctive smell and a diverse range of animals display extreme sensitivity to it. It has been reported that humans can detect 100 parts in a trillion.
In the soil, geosmin production is attributed to Streptomyces, a soil-dwelling bacteria which, when faced with unfavourable conditions grow spores which can be dispersed to new, more favourable conditions, enabling the bacteria to survive.
For many years geosmin production remained a mystery, but a defining moment arrived with the publication of the genome sequence of Streptomyces coelicolor A3(2) in 2002, a project driven by Sir David Hopwood, here at the John Innes Centre.
Scientists could now make the important link between gene and function. Subsequently, the lab of Prof Keith Chater, also at the John Innes Centre, showed that a single gene encodes the enzyme that catalyses the synthesis of geosmin, and Professor David Cane’s lab at Brown University, USA discovered the biochemistry underlying this process.
H – Hymenoscyphus fraxineus
Back in 2012 John Innes Centre researcher, Dr Anne Edwards recognised Ash dieback disease in ancient woodland in Norfolk and triggered a call to arms. The disease that had decimated 60 to 90% of ash woodland in Denmark was about to invade.
The fungus responsible, Hymenoscyphus fraxineus, is characterised by dark brown or orange lesions on ash leaves followed by wilting, lesions of dead cells on shoots and then diamond-shaped lesions on the stems. Finally, the crown of the tree dies back and the pathogen either kills the tree directly or weakens it to such an extent that it succumbs to other pests or pathogens.
In 2017, five years on from the discovery of ash dieback disease in Britain the future of the tree lauded as the ‘Venus of the Woods’ remained in the balance.
However, Professor James Brown’s group has recently been extending ideas from crop breeding to controlling the ash dieback fungus and is researching trade-offs between responses of ash to dieback and insect pests, including emerald ash borer, and strategies for promoting natural selection of durable resistance of ash to dieback.
I – Inoculations
Inoculation, using agrobacterium is one of the two main methods for delivering different DNA into a cell (the other being the particle gun).
Our Postdoctoral Scientist Erica Hawkins explains in her ‘What is plant transformation’ blog; “Agrobacterium is a naturally occurring soil bacteria, which has the unique ability to transfer part of its own DNA into plant cells. In the wild, transfer of some of the bacterial DNA causes rapid plant cell division, and the development of a plant tumour.
In the lab we harness the DNA transfer ability of Agrobacterium but remove the ‘tumour forming’ genes- instead replacing these with our genes of interest. We inoculate Agrobacterium containing our genes of interest, onto wounded plant tissue explants. The Agrobacterium then transfers the gene of interest into the DNA of the plant tissue.”
J – JBS Haldane
John Burdon Sanderson Haldane was employed by the John Innes Trustees for 10 years, from 1927 to 1937.
The eminent mathematical biologist, and co-founder of the ‘synthetic theory of evolution’, was employed by the John Innes Trustees, under the Directorship of Sir Daniel Hall, on a part-time basis whilst at the University of Cambridge. Unable to find a suitable person to lead the genetics research at John Innes, Hall convinced the Trustees to employ Haldane in an advisory capacity, to which Haldane firmly adhered, never once performing any experiments whilst at John Innes.
There is little doubt that Haldane’s contribution during those years ensured the continued development of genetics research at John Innes and maintained the foundations laid by William Bateson our first Director.
K- Knock-out mutants
A gene knockout is a genetic technique of rendering a gene inactive andis used to study the role of the gene in the organism.
These are used to uncover the function of genes in microbes. For example Professor Barrie Wilkinson has used deletions in genes to uncover proteins that maintain the production of the antibiotic formicamycin.
L – Light microscopy
At the John Innes Centre we have state-of-the-art bioimaging facilities, in both light and electron microscopy.
Our light microscopy equipment includes; conventional bright-field, phase, DIC (Nomarski), and fluorescence imaging, laser-scanning and spinning-disc confocals, with FRAP, FRET, FLIM and FCS capabilities and, to go beyond the diffraction limit, a Zeiss LSM880 Airyscan fast and an Elyra PS1 for SIM and STORM.
The team specialise in the imaging of plants and microbes but can also cater for a wide-range of imaging requirements for animal cells and material science. Recently our bioimaging team helped Dr Susan Schlimpert see the complex dynamics of cell division in Streptomyces, the first time this was possible.
M – Micron
The MICRON network showcases the wide variety of microbiological research taking place on the Norwich Research Park.
The Norwich Research Park encompasses scientists and projects here at the John Innes Centre, as well at The Sainsbury Laboratory, Quadram Institute, Earlham Institute and the University of East Anglia, making Norwich the UK’s leading site for microbiological research.
The network has been established to improve links between researchers and research bodies, helping to drive high quality research and open lines of communication between scientists both in Norwich, and across the wider research community.
N – Nodules
Nodules are lumps that form on the roots of plants, in particular legumes such as beans, lentils and peas. Root nodules form a symbiotic relationship with nitrogen-fixing bacteria and are our scientists are interested in studying this interaction.
One of the major factors that limit crop growth is the availability of nitrogen, but currently only bacteria and other single-celled microbes called archaea can take nitrogen from the air and fix it into a form that can be used by plants. The process carried out by these microbes is known as biological nitrogen fixation.
The Professor Ray Dixon group focuses on understanding nitrogen fixation in bacteria and its regulation in response to environmental cues. Legumes obtain nitrogen from symbiotic nitrogen-fixing bacteria, but cereal crops including wheat and maize, rely on the availability of fixed nitrogen in the soil. In many cases the addition of chemical fertilisers is the only way to provide crops with enough nitrogen to ensure a good harvest. Nitrogen fixation is an intricate and delicate process which requires a balance of numerous key components. Until now, achieving the right balance of these components has been a major challenge to the engineering of nitrogen fixation in cereal crops.
Nodules are also useful for other areas of scientific investigation. For example, Dr Myriam Charpentier uses root nodulation in response to nitrogen fixing bacteria as a model for observing nuclear calcium signalling in plants and how these signals are produced in response to environmental and biological stimuli.
Meanwhile Dr Janneke Balk’s group monitors iron delivery to the bacterial symbionts housed in root nodules, using an iron-responsive reporter they developed in the lab. They have used this to analyse mutants of the host plant Medicago truncatula to see if a particular plant gene is required for iron delivery to the symbiont
O – Oomycetes
Oomycetes are a group of microscopic organisms, sometimes known as ‘water-moulds’ and contain a number of plant pathogens.
He hypothesises that pathogens benefit from the host intercellular communication in two ways: by delivery of mobile signals from invaded cells into adjacent, ‘to-be-invaded’ cells to promote disease; and by gaining access to resources from surrounding non-invaded plant cells. To test this, Xiaokun uses the compatible interaction between the oomycete pathogen Hyaloperonospora arabidopsidis (Hpa) and its host, Arabidopsis.
P – Pseudomonas
Some microbes are good or bad, depending on their strain and environment. One of these such microbes is Psuedomonas which lives in soil and water.
These bacteria can be a bad guys, for example the species Psuedomonas syringae is a widespread plant pathogen which affects a range of fruit and vegetables around the world. It is responsible for tomato black spot, which causes yield losses due to reduced quality, and kiwi canker, a blight that has devastated kiwi crops across the world. Dr Jacob Malone is researching uses bacteria-killing viruses called bacteriophages to stop infection of Pseudomonas. Malone’s group, together with Folium are working on a project to harness bacteriophages as a natural biocontrol agent.
Dr Malone is also investigating how we can learn from these bad guys to our benefit crops, as they produce useful compounds such as antibiotics.
Pseudomonas doesn’t just infect plants, other strains such as Psuedomonas aeruginosa can cause infections in animals and humans, particularly in people with cystic fibrosis.
However, Pseudomonas can also be beneficial for example in helping plants fight of other infections. As Dr Malone explains another strain “Pseudomonas fluorescens is capable of suppressing some important fungal and bacterial infections in crop plants, including potato scab, powdery mildew and take-all, a disease that can devastate wheat yields. Dr Malone’s current research focuses on establishing exactly which of Pseudomonas’ characteristics are important for its agriculturally beneficial interactions in the soil microbiome”.
Q – Quinolone
Quinolone antibiotics are an important and powerful group of human medicines.
The increasing rate of antibiotic-resistant bacteria causing life-threatening infections, is a global threat.
Bacteria have multiple tools to resist the action antibiotics. We already know a lot about them, but we still don’t fully understand how and when microorganisms develop antibiotic resistance. Also, we don’t know how antibiotics, apart from killing bacteria, can have the opposite effect and “make” bacteria antibiotic-resistant.
Quinolones are antibiotics that inhibit topoisomerases (enzymes that modify the topology of DNA). It is been shown that quinolones can induce antibiotic resistance in bacteria, although the underlying molecular mechanisms are not yet clear.
However, scientists from the John Innes Centre, Quadrum Institute and the University of Birmingham have recently discovered a link between a major mechanism of antibiotic resistance and resistance to the disinfectant triclosan which is commonly found in domestic products.
R – Resistance
Resistance is a key aspect of a lot of the plant and microbial research we do at the John Innes Centre, where we are working on both disease resistance in crops and antimicrobial resistance.
Professor Tony Maxwell is working on antimicrobial resistance (AMR), which is the ability of a microbe to survive antibiotic treatment. AMR also covers other microorganisms like fungi, which can be problematic in terms of human infections
S – Streptomyces
Streptomyces are soil-dwelling bacteria that produce approximately two-thirds of the antibiotics in current clinical use.
Work on Streptomyces at the John Innes Centre dates back to 1968 and the launch of our Streptomyces genetics programme, headed by David Hopwood. In an interview in 2017 Professor Sir David Hopwood described what was known about Streptomyces at the time he began working on them; “When I started, somebody in the Botany School at Cambridge, a guy called Lewis Frost, said to me, here are streptomycetes, people don’t know whether they’re bacteria or fungi or something in between, why don’t you see if they have any genetics, because already at that time bacterial genetics and fungal genetics were clearly extremely different.”
This year another Streptomyces research breakthrough was achieved when time-lapse microscopy helped reveal a brake mechanism in the Streptomyces lifecycle.
T – Tulip breakage
With a history stretching back to 1910, we have a long history of plant and microbial breakthroughs at the John Innes Centre.
One of the most important for gardeners, came in 1927 when mycologist Dorothy Cayley, John Innes, showed that ‘breaking’ in tulips is caused by a transmissible virus. ‘Breaking’ describes colour variegation of the petals into splashes, stripes or lines distributed on a white or yellow background. This pretty effect was nevertheless a commercial disadvantage; many tulip growers wanted to ‘rectify’ their tulips to get ‘true’ colours.
Cayley’s work showed that colour ‘breaking’ was due to ‘virus or enzyme infection’, probably spread by aphid attack. Her experiments suggested the degree of breaking was proportional to the amount of infected tissue introduced and was published in the ‘Annals of Applied Biology’ in November 1928.
U – Undergraduate Summer School
Our International Undergraduate Summer School is a unique opportunity for undergraduates from all corners of the world to spend eight weeks at our internationally renowned research centre, partnering with fellow Norwich Research Park institutes; The Sainsbury Laboratory and The Earlham Institute. Students experience plant and microbial science, interacting with some of our world-leading scientists and gain an unrivalled insight into research, for your future career.
One afternoon each week is dedicated to training events that cover activities such as science communication, computer programming, field studies, advanced presentation skills, and discussions with students from previous years about career development.
The programme culminates in a two-day conference on the beautiful North Norfolk coast where students present and share their research.
V – Virus-like particles
His group use a synthetic biology approach to create synthetic virus-like particles and they have exploited a highly efficient transient expression system developed in their laboratory (the CPMV-HT system) to produce a range of pharmacologically active proteins.
Their work has been shown to be able to protect sheep against bluetongue virus (BTV) and has resulted in the filing of several patents.
W – Wheat blast
Wheat blast is a destructive disease of wheat in Brazil and other countries of South America caused by a fungus. In 2016, it appeared in Bangladesh causing devastation, and the following year it spread to India. The major wheat belts of India and China have warm, wet climates that enable proliferation of this disease making it a potential threat to food security in this region.
Rachel aims to identify sources of resistance to these diseases and develop new high-throughput marker assays to enable plant breeders, both in Brazil and elsewhere, to incorporate high levels of resistance to these two diseases into future wheat varieties.
X – Xylella fastidiosa
Xylella fastidosa is not present in the UK and for many years the bacterium was restricted to the Americas where it caused serious disease outbreaks in crops such as citrus, coffee, grapevine and peach.
However, in 2013, Xylella was identified in diseased olive trees in the south of Italy. Since then, the disease has killed millions of trees and the area affected has expanded, despite efforts to contain the outbreak. The bacterium has also been identified to be causing disease outbreaks in ornamental and native species, such as lavender, oleander and rosemary, in France and Spain, leading to the European Commission describing it as “one of the most dangerous plant bacteria worldwide, causing a variety of diseases, with huge economic impact for agriculture, public gardens and the environment.”
To help combat it, we are playing a key role in BRIGIT, which aims to build the UK’s capability to prevent establishment of vector-borne plant pathogens and to increase our preparedness to respond should they be introduced.
Y – Yeast
Fission yeast is a model organism, in the same vein as Arabidopsis.
As such, we study fission yeast for a number of reasons, for example helping determine how cells use geometrical information to control key developmental processes such as the coordination between cell growth and cell division.
Z – Zymoseptoria tritici
Zymoseptoria tritici is a fungal wheat pathogen that causes he foliar wheat disease Septoria tritici Blotch, which causes significant yield losses in temperate regions worldwide.
Postgraduate Researcher Amber Hafeez is working on finding resistance to Septoria tritici Blotch and aims to identify variation in the response of bread wheat, Aegilops tauschii.