A – Z of Plant Science
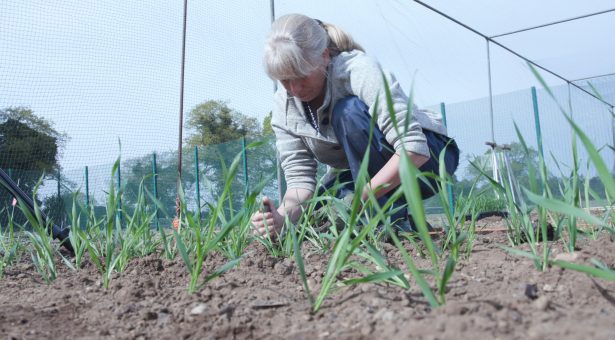
With plant research stretching from the genetic to the gigantic, we thought it would be worth creating an A-Z of some of the key pieces of work being done here.
A – Arabidopsis
Arabidopsis is an unassuming member of the brassica family, which has had an immeasurable impact on the study of genetics.
Throughout science, researchers use model organisms which are extensively studied to understand specific biological processes, with the expectation being these discoveries can shed light on the workings of other organisms. The archetypal and best-known model organism is the guinea-pig, thus the colloquial phrase; ‘to be a guinea-pig’. Other model organisms include mice, zebra-fish and arabidopsis, which possesses many qualities which make it a successful model organism.
Firstly, Arabidopsis is small and low maintenance. It also rapidly produces large numbers of offspring enabling scientists to see comprehensive effects of their interventions very quickly.
Furthermore, Arabidopsis has the ability to both cross-fertilise and self-fertilise. As such, scientists can reap the rewards of both reproductive strategies and a whole line of identical Arabidopsis plants can be produced from just one individual. Plus, if any harmful deleterious mutations should arise, these can be promptly bred out of the strain.
B – Brassica
Brassica’s are one of the species we specialise in at the John Innes Centre, with a number of research groups working on different crops, including broccoli and oilseed rape.
Why do we focus on brassicas? Brassica species encompass a diverse range of economically important crop species worth in excess of $20 billion worldwide and Oilseed rape (Brassica napus) is the second largest crop within the UK.
Our Brassica research programme targets economically important traits such as germination and establishment, flowering time, pod shatter resistance and pest and pathogen interactions. Ultimately our goal is to understand seasonal and environmental effects on developmental transitions in Brassica crops, and to use this information to improve reliability, yield and quality.
C – CRISPR/Cas9
CRISPR/Cas9 is one of the latest tools in a plant scientist’s toolbox, allowing efficient and accurate genome editing.
Through our Biotechnology Resources for Arable Crop Transformation (BRACT) platform, we have a long history of providing efficient crop transformation to the research community. CRISPR/Cas9 technology has allowed us to develop new targeted gene knockout resources, using which we have demonstrated efficient genome editing in barley and Brassica crops.
D – Developmental biology
Plant growth is shaped by the interaction between internal gene expression programs and the external environment.
The department of Cell and Developmental Biology looks at the integration of gene activity and external signals; how their effects unfold from molecular through cellular scales to produce macroscopic plant structures.
The fundamental knowledge we create is relevant to the broader scientific community as well as to agriculture. Applying this understanding of the genes and processes that control plant growth, their responses to seasonal and ambient temperature, symbiotic interactions and disease contributes to the improvement and performance of crop plants.
E – Epigenetics
Epigenetic changes to gene expression are changes that are heritable through cell division but do not change the DNA sequence. These changes are often triggered by environmental or seasonal factors.
Back in March, an ambitious five-year scientific investigation into how organisms remember environmental changes at a cellular level was awarded European funding.
F – Fluorescence
Fluorescence is the emission of light by a substance that has absorbed light or other electromagnetic radiation, which we use to try and answer key questions in fundamental biological research.
For example, Professor Robert Sablowski’s group use fluorescence imaging of proteins to study how regulatory genes coordinate cell division and cell growth when plants grow new organs.
G – Genome Editing
Though Genome Editing sounds like the same thing as Genetic Modification, they mean slightly different things.
While early Genetic Modification techniques allowed scientists to insert novel genes directly into the plant genome, thereby speeding up the breeding process, where in the genome they were inserted was random and unpredictable. Thanks to technological advancements, such as Crispr/CAS9 Genome Editing now allows us to precisely open-up the genome at a known desired location to introduce new traits.
H – High-iron crops
Leafy vegetables, pulses, grains and potatoes are rich in iron, but this is not always bioavailable for human nutrition. Unfortunately, plants also contain high levels of anti-nutrients, such as polyphenols in tea and phytic acid in grains, which severely inhibit iron uptake.
In the UK and many other countries, wheat flour is fortified by adding iron salts or iron powder to help combat iron deficiency. Low iron intake can lead to iron deficiency anaemia which is a global health problem. According to the World Health Organisation, iron deficiency anaemia affects up to 2 billion people, mainly women and children.
I – Infiltration (Leaf Expression Systems)
One of our research themes within our Molecules from Nature Institute Strategic Programme is working towards enhancing and further developing our capacity to produce large amounts of valuable proteins and products very rapidly in the leaves of tobacco plants (Nicotiana benthamiana) via infiltration.
This “transient expression” system has many uses in our research, and great potential as a new manufacturing process and can rapidly produce proteins that are derived from disease-causing viruses but are themselves harmless.
When introduced into mammals and humans, these plant-produced proteins induce the production of antibodies which provide immunity against the virus from which they were derived. We and others have already shown that the system can be used to produce vaccines against bluetongue, seasonal and bird flu, and polio.
J – Janaki Ammal Scholarship
We are proud to offer Janaki Ammal Scholarships to overseas postgraduate research students, who are nationals of eligible developing countries, to help enable them to study for a PhD degree at our institute.
Janaki Ammal (1897-1984) was one of the first women from India to obtain a PhD in Botany (Michigan USA 1931). She worked at the John Innes Horticultural Institution briefly in 1931 and 1935, followed by a longer period between 1940 and 1945. This led to the publication of the Chromosome Atlas of Cultivated Plants, which she wrote jointly with the then Director, C. D. Darlington. She ultimately returned to India to continue a long and distinguished career in science.
Earlier this year, a new rose hybrid was named for Janaki by two Indian plant breeders, Girija and Viru Viraraghavan.
K – Knockouts
A key aspect of plant science is understanding the genetics of plants. A gene knockout, is when a gene is made inoperative. This allows researchers to decipher what role the gene may play in a plant, by drawing comparisons between knockouts and a plant with all the other genes the same. There are various ways that knockouts may be generated, such as mutagenesis and CRISPR/Cas9.
Knockouts are often used in reverse genetics, when researchers look to see how a plant changes as a result of particular sequences. This is in contrast to the forward genetics of classic genetics screens where researchers try to uncover the genetic basis to explain a trait in a plant. An example of reverse genetics, are the TILLING populations in wheat, brassica and other species that are managed through our RevGenUK platform.
L – Leafless and semi-leafless pea
In the late 1960s, many vining pea crops were being bypassed by the harvesters because of the slow throughput of the harvesting machinery. Throughout Europe there was a demand for crops with acceptable yields but less stalks and stems.
This research proved to be revolutionary for the pea industry; the enhanced standing ability led to reduced yield losses and increased the popularity of cultivated peas as a quality food and feedstuff, with the potential of reducing our reliance upon imports for plant protein.
The development of the ‘semi-leafless’ pea illustrates how physiological analysis can benefit a breeding programme and highlights how links between researchers, breeders and growers can help address crop problems.
M – Marple
The Dr Diane Saunders group have pioneered a revolutionary genomics-based pathogen surveillance technique called ‘field pathogenomics’ that uses the latest DNA sequencing technology to generate high-resolution data quickly for describing the diversity in a pathogen population directly from infected field samples. This information is essential to help breeders to develop wheat varieties that are resistant to the wider range of yellow rust isolates that they now find in the field.
Through international collaboration with CIMMYT and the Ethiopian Institute of Agricultural Research this technique has been further developed into a portable platform called Mobile And Real-time PLant disEase (MARPLE) diagnostics that is allowing scientists in Ethiopia to track the spread of individual strains of wheat yellow rust in near real-time. This in turn enables stakeholders to make immediate decisions regarding disease management within the current growing season.
N – Nitrogen fixation
One of the major factors that limit crop growth is the availability of nitrogen, but only bacteria and other single-celled microbes called archaea can take nitrogen from the air and fix it into a form that can be used by plants. The process carried out by these microbes is known as biological nitrogen fixation.
Cereal crops including wheat and maize, rely on the availability of fixed nitrogen in the soil. In many cases the addition of chemical fertilisers is the only way to provide crops with enough nitrogen to ensure a good harvest.
However, we are studying the mechanism engineering nitrogen fixation, along with colleagues in Chinawhich brings us one step closer to realising the goal of engineering a range of crops to fix their own nitrogen.
O – Organogenesis
Organogenesis and Plant Architecture, is one of the main themes of our ‘Genes in the Environment’ programme. The aim is to understand how plant cells use genetic instructions to produce particular physical plant structures.
We aim to develop a deeper understanding of how cells respond to genetic instructions as they form the organs of a plant, and identify useful variations in individual genes in wheat, barley and oilseed rape which can improve yield and yield stability
P – Purple tomatoes
Plants contain a huge array of natural chemical compounds and some of them are increasingly seen as ‘natural medicines’ able to provide protection against human chronic diseases.
The Martin lab have generated purple tomatoes with very high levels of specific phenolic compounds combining the use of transcription factors, biosynthetic genes and RNAi with the availability of natural tomato mutants.
Using purple, high-anthocyanin tomatoes they have shown that their inclusion in the diet of cancer-prone mice can extend life-span by 30%, resulting in worldwide recognition that anthocyanins are important health-promoting dietary constituents.
Q – Quantification
Precise and accurate measurement of plants, particularly in a field environment plays a vital role in our work towards genetic improvement of crops.
To that end, as part of our Field Experimentation facilities, we have almost 3ha of growing space on-site, which is currently home to our Phenospex phenotyping platform providing 24-hour laser scanning of plots grown in a farm environment.
Further afield we own 110ha at Church Farm, Bawburgh, where we will be bringing together lab and field research in one location to improve facilities for research in understanding how genes control plant growth in the field.
The Dorothea de Winton field station is set in 110 hectares of farmland, of which 20 hectares are used each year for field research on crop and non-crop plant species. The Field Station features two laboratories, climate-controlled grain storage, office space for six staff, meeting rooms and storage space for agricultural equipment.
We have a collaborative arrangement with The Morley Agricultural Foundation which allows us to do multi-site experimentation, 10 miles from the John Innes Centre.
R – Rapeseed
UK rapeseed growers are losing up to a quarter of their crop yield each year because of temperature rises during an early-winter weather window.
This figure emerged in new research by the John Innes Centre which identifies a critical period from late November to the Winter Solstice, December 21 or 22, where temperature has a strong link to yields.
The research, which appears in the journal Scientific Reports, reveals that a mere one-degree temperature rise in this volatile weather period costs UK rapeseed growers £16m in lost income six months down the line when the crop is harvested.
Based on analysis of climate and yield data, the team calculate that temperature variation during this critical time window can lead to losses of up to £160 million in the UK rapeseed harvest – about 25% of the total value.
S – Speed breeding
Technology first used by NASA to grow plants extra-terrestrially is fast tracking improvements in a range of crops.
Speed breeding uses enhanced LED lighting and day-long regimes of up to 22 hours to optimise photosynthesis and promote rapid growth of crops. It speeds up the breeding cycle of plants: for example, six generations of wheat can be grown per year, compared to two generations using traditional breeding methods.
By shortening breeding cycles, the method allows scientists and plant breeders to fast-track genetic improvements such as yield gain, disease resistance and climate resilience in a range of crops such as wheat, barley, oilseed rape and pea.
Recently scientists here, along with colleagues at the Earlham Institute, and Quadram Institute in Norwich and the University of Queensland in Australia have improved speed breeding techniques, adapting it to work in vast glasshouses and in scaled-down desktop growth chambers.
T – Triterpene biosynthesis
Professor Anne Osbourn investigates plant natural product biosynthesis and has developed a synthetic biology platform for rapid gram-scale production, using triterpenes as an example.
Terpenes are one of the largest and most diverse classes of plant-derived natural products and have a wide range of applications in the agriculture, pharmaceutical, food and manufacturing industries. These compounds have a high degree of structural complexity, making them inaccessible to organic synthesis or classical combinatorial chemistry.
The lab have characterised an extensive set of genes and enzymes for triterpene biosynthesis and are using this toolkit to engineer structurally diverse molecules so that we can investigate the relationship between structure and function. They aim to create new methods, platforms and technologies for the rapid discovery, synthesis and modification of triterpenes that would not otherwise be accessible.
U – Utricularia gibba
Bladderworts (Utricularia vulgaris) are a species of underwater plant, which use their shape to ready to suck in any unsuspecting water-dwelling creature that comes too close. Utricularia are found around the world, on every continent except Antarctica.
The Coen lab studies how Utricularia gibba modify their leaves along pre-existing frameworks to grow complex organs, U.gibba are a great model for studying how plants form complex leaf shapes because, it grows very happily in tissue culture, it has a small genome at 100 Mb and produces lots of offspring. They also have a long sprawling stem sporting hundreds of traps, which are a mere 1 mm in length and only two cells thick, so easy to fit under the watchful gaze of a microscope.
Our work involves some of the latest in computational and imaging techniques to reveal how these deadly, but beautiful, traps make themselves, showing how, from a tiny bundle of cells, fascinating growth dynamics occur to allow an intricate shape to form through growth.
V – Vernalization
In order to survive, plants have to flower at the right time, namely in spring, and not autumn, even if the autumnal conditions seem favourable, otherwise they would perish once the cold of winter sets in.
In order to only flower in the spring plants need to sense and then remember winter, a process known as vernalisation. But how do plants sense vital information such as temperatures to align flowering with the seasons?
Until recently, many researchers thought that fluctuations in monthly, daily, hourly temperatures were detected by a small number of dedicated sensors. But new research by the John Innes Centre reveals that plants combine the temperature sensitivity of multiple processes to distinguish between the seasons.
W – Wheat
Wheat is one of the most important global crops and is grown on more land than any other commercial crop. It currently provides 20% of total calories consumed by humans daily worldwide.
As the global population increases towards 10 billion people, with most increased consumption expected to occur in developing countries, it is anticipated that the world will need to produce 60% more wheat by 2050 to meet global demand.
Since it takes between 15 and 20 years for current research to improve wheat varieties grown in farmers’ fields, it is imperative that we act now to address problems facing us in the future.
As such, one of our four Institute Strategic Programmes, is dedicated to ‘Designing Future Wheat’. It spans eight research institutes and universities and aims to develop new wheat germplasm containing the next generation of key traits.
X – X-Ray Protein Crystallography
We use X-ray protein crystallography to reveal the atomic details of biological systems. This provides deep insights into molecular interactions, gene regulation, enzyme mechanism, protein function and protein evolution.
Along with Proteomics, Nuclear Magnetic Resonance and Small Molecule Mass Spectrometry, Protein Crystallography makes up our suite of Biomolecular Analysis technology platforms.
Y – Yellow Rust
Wheat yellow rust is a devastating disease that leads to significant reduction in quality and yield in susceptible cultivars.
It is caused by the fungus Puccinia striiformis f. sp. tritici (PST) and despite posing a major threat to global food security, the molecular mechanisms regarding host pathogen interactions in this pathosystem remain poorly understood.
Dr Diane Saunders’ research focuses on (re-)emerging plant pathogens that pose a significant threat to agriculture. Diane has a particular interest in the wheat rust pathogens, which are known as the “polio of agriculture”.
In one recent project, the group pioneered a revolutionary genomics-based pathogen surveillance technique called ‘field pathogenomics’ that uses the latest DNA sequencing technology to generate high-resolution data quickly for describing the diversity in a pathogen population directly from infected field samples. This information is essential to help breeders to develop wheat varieties that are resistant to the wider range of yellow rust isolates that they now find in the field.
Z – Zinc
According to the World Health Organisation, more than 2 billion people across the globe are suffering from dietary deficiency of mineral micronutrients, particularly iron and zinc.
Wheat is one of the staple food crops and therefore, a suitable candidate for biofortification, which is being worked on by Dr Janneke Balk’s lab, who are seeking a greater understanding of the mechanisms which control the transport and deposition of iron and zinc in the developing grain as this should allow similar effects to be achieved by exploiting naturally induced genetic variation. When combined with conventional biofortification and innovative processing, this approach should provide increased mineral bioavailability in a range of wheat products, from white flour to wholemeal.
Postgraduate Researcher Camilla Stanton is also working on zinc in the group of Professor Tony Miller.
Camilla’s looking for novel components of the zinc homeostasis network in the model plant species Arabidopsis thaliana through a genetic screen. A deeper understanding of how plants regulate zinc levels could help breeders produce crops with improved zinc content in the future, and consequently combat zinc malnutrition in the human population.
Camilla is also interested in exploring zinc dynamics in response to various stimuli by using genetically encoded zinc-specific Fluorescence Resonance Energy Transfer (FRET) sensors.