Using genetic technologies in plant and microbial science
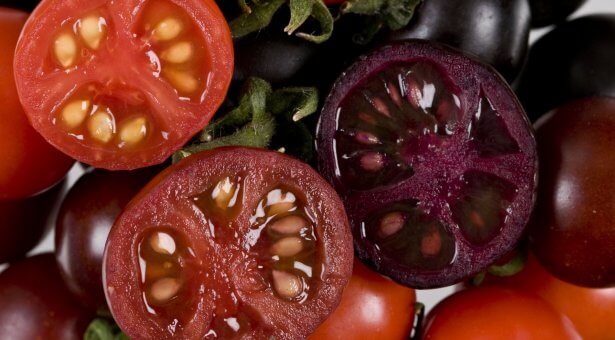
For over 100 years, genetics has been a key thread running through our research at the John Innes Centre.
Now, with the rapid development and expansion of sequencing technologies, we have an explosion of information available to us. The majority of crop plants have now had their genomes sequenced and so more than ever, genetics is a vital component of our work.
There is a huge amount of genetic variation in crops, which result in different characteristics, also known as traits, which influence things such as disease resistance, improved nutrition, reduced seed loss and increased seed size. We study these traits with an eye on helping ensure the future includes: healthy people, healthy plants and a healthy planet.
Why we’re interested in genetics
We use genetic techniques to alter the DNA of the plants and microbes that we study at the John Innes Centre.
This allows us to test what an individual gene or DNA sequence does and helps us discover which genes correspond with which traits, as well as how changes to the genes influence how the organism develops, grows, and interacts with its environment.
This approach can improve our understanding of how an organism’s genetics contributes to its form and function.
Our researchers can also use genetic techniques to introduce genes that convey specific traits including resistance to pests, greater tolerance to drought or better nutritional value. This can help reduce the need for chemical inputs in agriculture, reduce our carbon footprint, and create crops which are more resilient to climate change.
Traditional plant breeding
Traditional plant breeding centres on selecting desirable traits from different parents.
This can be achieved by crossing two individual plants with desirable characteristics and selecting for the desired combinations in the offspring. These traits could include things like yield, flavour or appearance.
Due to the length of time it takes to grow each generation of new plant and achieve the combination of traits required from each parent plant, this approach can take decades before you have a plant with the required traits. You are also limited to selecting those traits which appear in the gene pool.
Mutation breeding
Since the 1920s plant breeders have used a technique called mutation breeding – where new genetic variation can be induced by deliberately causing mutations.
Mutation breeding involves exposing seeds to either chemicals or radiation to introduce changes randomly throughout the genome –hundreds of thousands of random mutations are inserted in the genome, and any plants which have desirable traits introduced are then selected for breeding.
This provides access to a greater range of genetic variation than traditional breeding programmes.
However, mutation breeding is inefficient and can still take a long time to achieve desired results. This is because you are introducing tens of thousands of other random mutations that you may not want as well as introducing the few changes you do want.
To remove these unwanted changes, you need to take the offspring and ‘backcross’ it to the starting parent over several generations until you end up with just the required new gene, meaning the technique is labour and time intensive.
Historically, many of our modern crops have been produced through mutation breeding.
Modern breeding techniques
New genetic techniques enable us to make changes to an organism’s DNA, by introducing new genetic sequence or by making small and precise changes to the existing genetic sequence. This can be done without also mixing thousands of parental genes as in traditional breeding or inducing thousands of unwanted mutations as in mutation breeding.
Genetic modification
In the 1980’s a technique was developed where a genetic sequence, or piece of DNA, is introduced directly into an organism, giving it a new trait. This DNA could originate from the same species (cisgenic) or come from a different and non-sexually compatible species (transgenic). The term Genetically Modified Organism (GMO) applies to both situations.
Genetic Modification allows researchers to introduce gene(s) of interest, much more quickly than they can when using traditional or mutation breeding. One example of research of this type at the John Innes Centre is a recent series of field trials we ran on genetically modified wheat, to accumulate extra iron making it more nutritious and able to have a big impact on public health.
Gene editing
More recently, genome editing techniques have been developed. These allow us to make a specific edit to DNA in a targeted way – without adding in a new genetic sequence. Specific genes can be removed or changed, with small, targeted changes at a known location in the genome. This allows us to reach the same end point as traditional breeding, but more quickly and without the need for back crossing.
What we call gene editing is not just one type of technology – there are several different types. CRISPR-Cas9 is the most well-known gene editing method, particularly after it’s invention won the Nobel Prize in 2020, but there are other technologies for gene editing as well.
Gene editing is different from genetic modification because it is very targeted, the change made is at a specific place and there is no foreign DNA added into the final organism.
At the John Innes Centre, we are investigating the genes involved in seed pod formation. Ideally for farmers, seeds should stay in the pod until harvest but premature pod shatter results in massive loss of yield in oil seed rape. Our researchers are homing in on the genes that might play a role in pod shatter, and by disrupting them using gene editing, we can see the impact on the plant and whether certain genes are involved.
Meanwhile, in Streptomyces, a bacteria used to produce antibiotics, we have used gene editing to create a new strain of Streptomyces formicae bacteria which over-produces the medically promising super-bug targeting formicamycin antibiotics.