What is Protein X-Ray Crystallography?
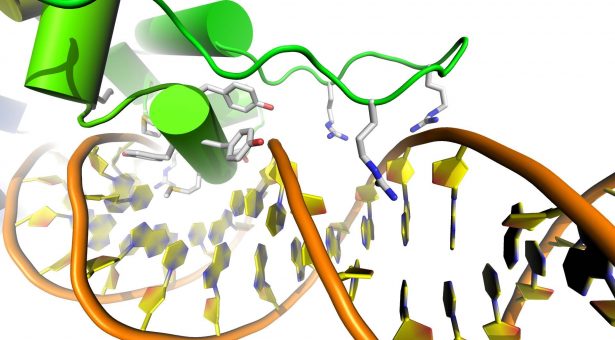
Professor Dave Lawson manages our Protein X-Ray Crystallography technology platform.
We sat down with him to ask what protein crystallography is, why it’s useful and how he got into this field of research.
“Protein Crystallography is a form of very high-resolution microscopy, which enables scientists to “see” at atomic resolution. It allows us to see beyond the capabilities of even the most powerful light microscope.
Proteins are at the heart of all biological processes and being able to see the fine details of their structures can be extremely informative. For instance, protein crystallography might help you to understand why a mutation in a protein has a drastic effect.
Alternatively, it can be used to give you information on the active site of an enzyme, allowing you to design molecules, which could target that area of the enzyme and provide the basis for a new drug.
In order to do Protein Crystallography, you first and foremost need to have a crystal to analyse.
To get your crystals, it’s a numbers game, you mix the target protein with hundreds of different chemical cocktails, in the hope that one or more of these will give you a crystal. Basically, you’re trying to persuade your protein molecules that are floating around in a solution to form an ordered crystal.
Thankfully we have a robot to setup the crystallisations for you – otherwise you would have to do a lot of pipetting… If you’re very lucky, your initial “hits” will be big enough to analyse straightaway, but often we then need to optimise the conditions to get suitable samples. If you can see the crystals with the naked eye, they are considered big crystals. A single protein crystal can contain around a billion molecules.
Once you get a good crystal, using a microscope you fish it out in a very small amount of liquid with a special loop, before plunging it into liquid nitrogen.
We perform the data collection on protein crystals at cryogenic temperatures, so that the molecules are frozen, this helps to give a sharper “picture”. As an added advantage, the crystals are less prone to radiation damage from the X-rays.
Once they are frozen, the crystals are transferred to a shipping dewar, and collected by a courier, who transports it to Diamond Light Source in Oxford. Diamond is a type of particle accelerator called a synchrotron that produces high intensity X-rays.
We work with Diamond Light Source as part of a consortium of labs based here at the John Innes Centre, as well as scientists at the University of East Anglia and the University of Essex, through which we get access once or twice a month.
What’s really cool is that while the actual process is taking place 150 miles away, we can control it all remotely. Webcams give us a view into the lab at Diamond, and from here we can select which samples are analysed, instructing the robot arm to pick them up one at a time and transfer them to a sample holder. You then need to precisely line up each crystal with the X-ray beam. Then you are ready to take the measurements.
In the experiments, we record a series of X-ray diffraction patterns, which with a good crystal, will appear as a collection of spots on the detector. The problem you have with X-rays, unlike with light microscopy, is that there is no equivalent of the lens to focus the image. In crystallography, you have to “focus” the image in silico. We do this by measuring all the spots on the diffraction patterns, and the computer software converts these into a 3D image of your protein.
All the atoms in a molecule are surrounded by a cloud of electrons, which effectively define its shape. In X-ray crystallography, the X-rays are diffracted by the electrons, so it is this cloud of electrons that we see, and it is referred to as an electron density map. The extent to which the crystal diffracts, determines what sort of resolution you can get in your image. With very good crystals, you can get down to seeing individual atoms.
At this point, you have a kind of 3D jigsaw puzzle to solve in order to determine the structure of the protein. Usually we know the amino acid sequence of the sample we have crystallised – we essentially solve the puzzle by fitting these amino acids into the electron density map. With good data, much of this can be automated, but you need to manually check it – and this is the fun part.
Recently we have been working on a collaboration with Professor Tony Maxwell, looking at the biosynthesis of a bacterial toxin that inhibits DNA gyrase, which is a validated drug target. Over the past 2-3 decades there has been a lot of literature about this toxin, but until recently it wasn’t clear exactly how it was made. So, we solved the structures of two protein complexes involved in producing the toxin, which turned out to be very enlightening, providing a 3D framework for understanding much of the previous data.
People think protein crystallography is hard, but thanks to many advances in equipment and computer software over the last few years, it is more straightforward than ever. Getting good crystals remains the bottleneck, but with automation and robotics, you don’t need as many good crystals as you once did, and they don’t need to be as big. Also, once you have crystals, we can analyse them very quickly.
As a child I had interests in nature and wildlife, so I always wanted to study biology and chemistry. I did a biochemistry degree and then went on to do a PhD with my undergraduate tutor in Sheffield, who was an X-ray crystallographer, so I sort of followed in her footsteps.
After my PhD, I was a Postdoc at the University of York for six years before coming to the John Innes Centre, where I was studying the structures of industrial enzymes for a company based in Copenhagen. These were the sort of things that might go into your washing powder or dishwasher tablets. In particular, I worked on a type of enzyme called lipase, which was used to break down fatty stains on clothing, and amylase, which breaks down the starchy stuff on your dinner plates.
As the name suggests, the platform mainly uses X-ray crystallography to resolve the structures of proteins, but recently, with the help of the Bioimaging platform, we have started to use the technique of cryoelectron microscopy as well. But that’s another story…”